III-Nitride Materials
and Devices Group
The University of New Mexico
Lab Capabilities and Research Projects

Lab Capabilities
Our research lab contains a Veeco P-75 turbo-disc metal organic chemical vapor deposition (MOCVD) system for epitaxial growth of III-nitride materials. III-nitrides consist of the binary materials GaN, InN, and AlN, and the ternery materials InGaN, AlGaN, and AlInN. III-nitrides exhibit direct band gaps over the entire visible spectrum and in theory also provides access to deep UV and IR emitters. III-nitrides are the preferred materials for visible optoelectronics (light-emitting diodes and diode lasers) and are finding new applications in power electronics, intersubband optoelectronics, photocatalysis, and photovoltaics. Also available in our lab or at CHTM are nanofabrication facilities and optical and structural characterization facilities. Our group also works closely with the Center for Integrated Nanotechnologies (CINT) and Sandia National Laboratories.

Nanoscale Selective-Area Epitaxy
We are using selective-area epitaxy to realize a variety of nanoscale features on sapphire, silicon, and GaN substrates. In this process, nanoscale openings are patterned into a dielectric material deposited onto a substrate. These features can be patterned in a well-controlled manner using interferometric or electron beam lithography, permitting intelligent deisgn of the feature size, periodicity, and fill factor. Standard or pulsed MOCVD growth is then used to grow nanoscale features out of the openings in the dielectric. Both axial and core-shell heterostuctures can be realized. This technique leads to the reduction or complete elimination of threading dislocations, results in increased effective active region area, and enables nonpolar quantum wells on foreign substrates. In addition, the potential for strain relaxed materials on foreign substrates may lead to improvements in solid-state lighting and generate novel laser and transistor structures.

High-Speed nanoLEDs Using Core-Shell Nanowires
Sponsored by DARPA YFA: As conventional wire-based electrical interconnects approach capacity limits, high-speed photonic devices are necessary components for the development of short-range optical interconnects. However, the requirements for the devices in such a system are very demanding. In particular, these devices would need to operate with high bandwidths (BWs) (> 60 GHz), exhibit low energy consumption (~10 fJ/bit), and be produced using a low-cost, silicon-compatible process. Since the BW of conventional directly modulated laser diodes is limited to several tens of GHz and their lowest reported power consumption is > 250 fJ/bit, novel photonic devices will likely be required to meet the demands of optical interconnects. Nanoscale light-emitting diodes (nanoLEDs) have the potential to achieve ultra-high modulation BWs (> 100 GHz) and low power consumption. These devices are designed to operate below the lasing threshold and utilize the enhanced spontaneous emission rates achievable in highly resonant cavities (i.e., the Purcell effect) to increase the BW beyond previously attainable levels. Plasmonic nanocavities provide a path toward overcoming diffraction-limited mode volumes, permitting ultra-high BWs with nanoLEDs. We have developed novel selective-area nanoscale epitaxy that results in ordered arrays of defect-free GaN nanowires (NWs) with controllable geometries and well-defined positions on either sapphire or silicon substrates. We are utilizing these NWs to fabricate metal-coated InGaN/GaN nanoLEDs, where plasmonic effects can reduce the mode volume far beyond the diffraction limit, allowing for ultra-high-speed (>100 GHz) direct modulation. We are exploring both core-shell and axial quantum well geometries. Initial device development is occuring on sapphire substrates, while the ultimate goal will be to realize high-speed nanoLEDs on (001) silicon. The success of this project would pave the way for more manufacturable, easily scalable, and higher-speed photonic systems, opening opportunities in optical communications, supercomputing, and chemical/biological sensing.

Nonpolar Nanowalls for High-Efficiency Green LEDs
Sponsored by NSF ERC for Smart Lighting: This research is part of the Smart Lighting Engineering Reserach Center at Rensselaeer Polytechnic Institute and is focused on developing a solution to the critical issues of “green gap” and “efficiency droop” in solid-state lighting by utilizing large effective active region areas, eliminating polarization, and reducing strain and defect densities with nanoscale selective-area growth of nanowall LEDs. The project goal is to develop fully-packaged prototypes of nanowall LEDs, and explore efficient integration with sensors and driver electronics. Thus far we have developed a process for defect-free GaN nanostructure templates and patterned nanowall growth, demonstrated the basic concept of core-shell devices by realizing electrically injected core-shell structures, achieved nanowall growth within LED mesa patterns, demonstrated photoluminescence from nanowalls up to ~530 nm, and developed a fabriaction process for electrically injected LEDs. Future work is focused on optimizing growth and fabrication conditions and exploring alternative nanostructure gemoetires, such as pyramids and wires.
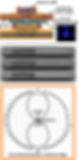
Nonpolar GaN-Based VCSELs
Research performed by Prof. Feezell at the Unviersity of California Santa Barbara: Recently, GaN based vertical-cavity surface-emitting lasers (VCSELs) have attracted significant attention as potential light sourcesfor display applications, high-density optical data storage, high-resolution printing, and bio-sensing. VCSELs offer several distinct advantages over conventional edge-emitting lasers, including lower threshold currents, single longitudinal mode operation, wafer-level testing, circular and low divergence output beams, and the ability to formdensely packed, two-dimensional arrays. Nonpolar InGaN quantum wells (QWs) exhibit several characteristics that are advantageous for VCSELs, including low transparency current densities, linear gain vs. current characteristics, high peak gain, and anisotropic gain within the QW plane. The former characteristics allow for designs with lower threshold currents and/or higher light output powers. The latter characteristic ensures that the polarization of the lasing mode is consistently aligned along a particular crystallographic direction. Specifically, in this project we showed that the lasing mode of nonpolar m-plane VCSELs is consistently linearly polarized along the (11-20) a-direction. In the future, this unique feature might be used to fabricate arrays of GaN VCSELs which are inherently polarization-locked and may be attractive for a variety of applications, including displays, sensors, pico-projectors, coherent detection systems, and communications. This is in contrast to most VCSELs, in which the circularly-symmetric cavity and isotropic in-plane gain result in a randomly polarized lasing mode. In this work, we demonstrated for the first time a nonpolar m-plane GaN-based VCSEL. We developed a process for nonpolar GaN-based VCSELs that utilized photoelectrochemical (PEC) undercut etching of an intracavity-embedded InGaN sacrificial layer to remove the substrate and expose the backside of the cavity. This approach addressed two significant challenges that hinder the practical manufacturing of GaN-based VCSELs: fabrication of high-reflectance distributed Bragg reflectors (DBRs) and precise control of the cavity length. The structure was grown on a free-standing m-plane GaN substrate using atmospheric-pressure MOCVD, contained 5 InGaN (7 nm) QWs with GaN (5 nm) barriers, a 15 nm Mg-doped AlGaN electron blocking layer, and top and bottom dielectric DBRs. An additional region of 3 InGaN (7 nm) quantum wells with GaN (5 nm) barriers was embedded in the n-GaN beneath the active region. After flip-chip bonding to sapphire, this region was laterally undercut using band-gap-selective PEC etching to separate the free-standing nonpolar GaN substrate from the devices. Using the above process, electrically-injected, single-longitudinal-mode nonpolar m-plane VCSELs were achieved with a room-temperature lasing wavelength of 411.9 nm, a full-width at half maximum (FWHM) of 0.25 nm, and a peak output power of 19.5 μW under pulsed conditions. The polarization direction of the VCSELs was consistently oriented along the (11-20) a-direction. The degree of polarization increased from 0.13 below threshold to 0.72 above threshold.
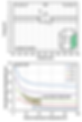
Intersubband Absorption With Nonpolar GaN/AlGaN
III-nitrides are currently employed in a broad range of visible-light applications, including solid-state lighting, displays, and high-density optical data storage. Beyond these well-established applications, these materials also provide a unique opportunity to realize higher-performance infrared devices based on intersubband transitions (ISBTs). III-nitrides exhibit large conduction band offsets (1.9 eV for AlN/GaN), short carrier relaxation times (170 – 370 fs), large longitudinal optical (LO) phonon energies (~90 meV), high-energy remote lateral valleys (>2 eV above gamma), and environmental stability. These properties provide several advantages over conventional materials used for ISBTs, including operation in the important telecommunications bands, higher operating temperatures, and the potential for high-speed devices based on resonant tunneling, such as THz quantum cascade lasers (QCLs). Thus far, investigations of III-nitride devices based on ISBTs have primarily focused on the conventional polar c-plane. However, devices fabricated on the conventional polar c-plane are plagued by spontaneous and piezoelectric polarization, which significantly compromise their performance and complicate the device design space. Specifically, these structures contain polarization-related electric fields that reduce the ISBT strength and limit the range of accessible wavelengths. Furthermore, the design of more advanced devices that require engineered carrier relaxation times to achieve population inversion, such as QCLs, is rendered difficult. Conversely, nonpolar orientations are free from polarization effects and promise to elicit the full potential of III-nitrides for applications based on ISBTs. In this work, we have compared the theoretical optical properties of intersubband transitions for polar III-nitrides, nonpolar III-nitrides, and conventional GaAs. We calculated and examined the peak transition wavelengths, dipole matrix elements, and absorption spectra as a function of quantum well thickness for single quantum well structures on each platform. We showed that the absence of polarization-related electric fields in nonpolar III-nitrides simplifies device design and facilitates clear performance advantages over conventional polar III-nitrides, including access to a wider range of absorption wavelengths, a several-fold increase in the dipole matrix element, and higher absorption probability. Compared to conventional GaAs-based structures, nonpolar III-nitrides exhibit a somewhat lower absorption probability but allow for a significantly wider design space, permitting devices operating at wavelengths that are unattainable using GaAs. (J. Appl. Phys. 113, 133103 (2013)).